Last month, the International Society for Evolution, Ecology, and Cancer Conference (ISEEC) took place in Hinxton, UK, at the Wellcome Genome Center. It was the second ISEEC I’ve been to, the first being held in Tempe, Arizona in 2017. It’s a unique conference in that it focuses on cancer as an evolving organism, taking into account the environment and conditions inside the body upon which selection acts. The speakers run the gamut from clinicians, physicists, mathematicians, to ecologists - and everything in between. Despite the range of disciplines, everyone who participates is focused on the singular goal of better understanding cancer through the lens of evolution. It is always exciting to see the latest developments in evolutionarily-driven therapies for the disease, and this year was no exception.
There was an undeniable emphasis on mathematical modelling this year across the conference, with even a full section dedicated to it hosted by Trevor Graham. The other sections were cooperation and conflict in multicellularity, cellular competition, cooperation and cancer, evolvability and adaptation, and transmissible cancer. As the theme of this blog is on mathematical oncology, I’ll focus on summarizing the math modelling section. Within this section of the conference, there was a common thread of cooperation and conflict, but spatial organization and environmental constraints were frequently mentioned by the speakers. First up was Diana Fusco, and her talk was titled: “What can microbial colonies teach us about spatial intra-tumor heterogeneity?”
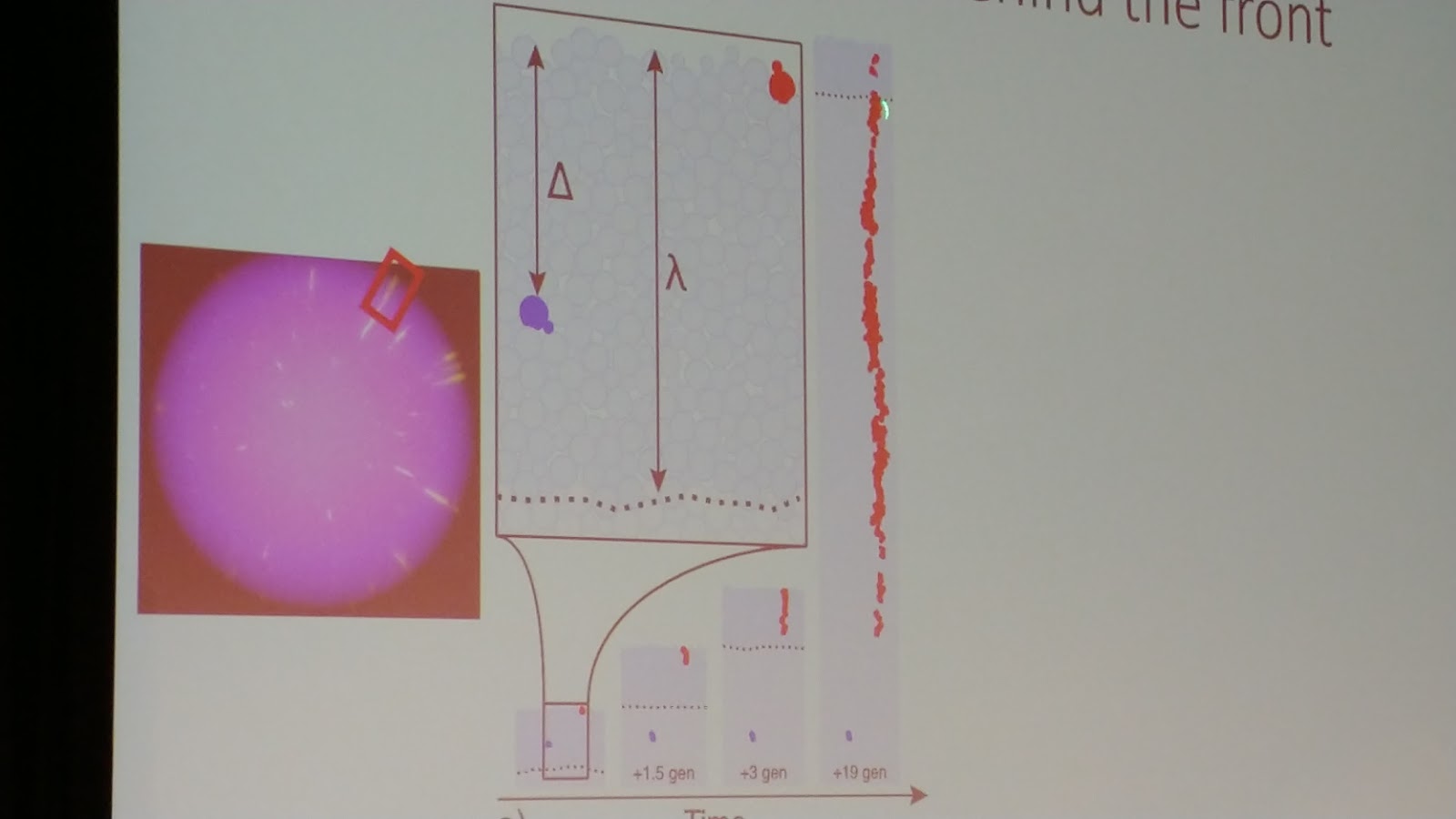
Diana is based at the University of Cambridge, and her work combines experimental microbiology with spatial modelling and statistics. Her talk was specifically on how microbial colonies can be used as analogs for intratumor heterogeneity. Spatial structure limits the ability of populations to grow indefinitely, but in well-mixed, exponentially growing populations, the frequency of mutation alleles follows a power law distribution. Her lab repeated the famous Luria-Delbruck experiment in
E. coli (mutations are pre-existing in a population, not generated as a result of selection itself), and then used modern sequencing techniques to show that this law holds true (
link to her paper on this topic). But they went a step further, and found that when
E. coli are grown as a colony on a petri plate, far more ‘jackpot’ mutation events are produced than when grown in well-mixed environments. Just like winning the genetic lottery, these jackpot events result in massive enrichments of high-frequency clones. Mutants at the resource-rich edge of range expansions can generate whole sectors of clones, a phenomenon also known as gene-surfing. Further, Diana’s lab found similar results in silico by replicating the experiment using an ‘Eden’ simulation (cells on a square lattice that can only reproduce into empty, neighbouring squares) and in 3D models.
Diana’s experiments serve to reinforce the idea of controlling cancer, rather than trying to eradicate every last cell in a tumor. They turned to an engineered strain of
S. cerevisiae that stochastically switched fluorescent marker expression at a rate of 1.6 x 10^-3 per cell division. During growth of a yeast colony, her group saw that most mutants actually go extinct - these pockets or ‘bubbles’ of mutant cells become trapped and outcompeted by wild-type cells, effectively becoming sealed off from the leading edge. If these mutants have a growth cost associated with them, it’s unsurprising that they can’t outgrow the wild-type. But when high doses of antibiotics are used, resistant mutants are released from their pockets. Whatever costs are associated with their growth are negligible compared to the wholesale destruction of their wild-type competitors. At intermediate levels of antibiotics, however, the whole population is suppressed without releasing those resistant clones - similar in principle to adaptive therapy for cancer. Diana’s group used microfluidics to see that the clone frequency distributions hold true regardless of dimensions, growth profiles, or even the mode of cell division.
Katerina Stankova: Cancer therapy: Changing the game
The next speaker was Katerina Stankova, who is based at Maastricht University in the Netherlands. Her talk was titled “Cancer Therapy: Changing the game” - appropriately, her research focuses on Dynamic Game Theory. She applies this theoretical framework to cancer treatments to design better, evolutionarily-enlightened therapies. There is a pressing need for this; existing treatment protocols do not consider evolution or game theory in their dosing schedules. Currently, in order to treat metastatic cancers, drugs are applied at the maximum tolerated dose (the highest dose that the patient can accept without toxic or dangerous side-effects) continuously or in a cyclical, repeated fashion. Doctors will then assess whether there’s been a response in tumor size, and decide on the next treatment based on toxicity or regrowth of the tumor. Disturbingly, despite this frequently-used protocol for treatment, there has been no increase in time to progression (TTP) for late-stage, metastatic cancers over the past 50 years (according to a World Health Organization report in 2018).
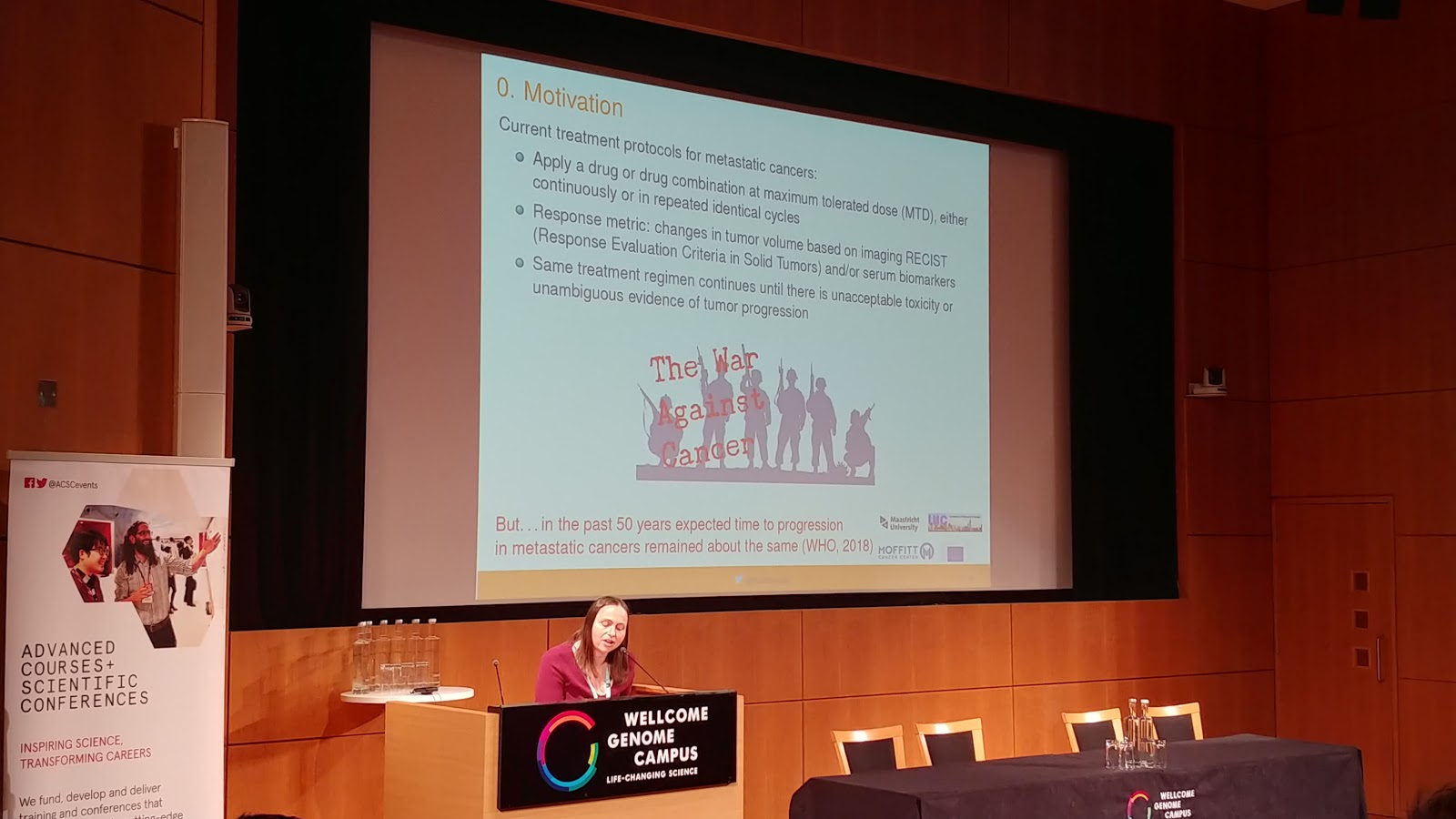
But times are finally changing, as Zhang et al. (2017;
link) published the first clinical trial based on game theory using adaptive therapy (changing the dosages of drugs based on the growth rate of the tumor). In castration-resistant metastatic prostate cancer, they designed a model using competing cancer ‘species’ to inform the clinical trial. By doing so, they were able to push back the median TTP of this form of prostate cancer from 16.5 months to 27 months or longer. The game theory rationale behind this trial was published as well (You et al., 2017;
link). Importantly, Katerina revealed that cancer therapy plays a ‘leader-follower’ type game - also known as a Stackelberg game. The leader is the physician administering the drugs, while the cancer cells are followers, capable of using a diversity of strategies to outplay the ‘rational’ doctor. According to a review recently published by Katerina (Stankova et al. 2019;
link), this results in the physician using a fixed strategy against an adaptive, ever-evolving enemy - and soon, the cancer takes over as the leader, with the physician hopelessly trying to play catch-up.
According to Katerina, there are different optimal strategies to consider based on the doctor’s end goal for the cancer. These could include objectives like minimizing the size of the tumor, keeping the size of the tumor stable, or even minimizing the number of resistant cells altogether. Importantly, physicians should be taking lessons from agriculture - namely, studying how farmers use resistance management plans when they use pesticides on crops. This is a hard-learned lesson designed to minimize the ability of pests to out-evolve their ‘static’ chemical-based selective pressures. Additionally, physicians should be analyzing and reporting on treatment failures, so they can learn why the cancer was able to advance in spite of their therapy.
About the Author:
Alex May is an evolutionary biologist with an interest in examining the emergence of cooperation across life and studying the consequences when it breaks down. His previous work included elucidating the role of cheating bacterial symbionts in the rhizobia-legume mutualism, examining the benefits of multicellularity in cancer cell clusters via artificial selection, and developing the fermented drink ‘Kombucha’ as a model of social behavior in yeast and bacteria. One of his main goals is to better communicate science to the general public, and has developed several educational ‘apps’ for this reason. Right now, he is working in industry on biomaterial research, but maintains links to his former supervisors and collaborates with them as much as he can. He is also hoping to collaborate with new researchers to design better tools (such as apps) for science communication.
Twitter:
@alexnmayhem
Game downloads:
https://www.indiedb.com/members/torerane/games